Anatomy and Physiology
Tardigrades may be tough, but they are tiny. In fact, their tiny stature may contribute to their toughness. There are more than 1000 species of tardigrades, ranging in size from less than 100 µm (1/10 of a millimeter) to just over 1 millimeter in length (Gross et al., 2015). Most species are less than half a millimeter long when fully grown. Their bodies are generally proportioned like little grains of rice: they are about 3 times as long as they are wide, though this ratio varies between and within species (Figure 1). Their small size and roughly cylindrical shape give them a relatively low surface area in proportion to their volume. This low surface area minimizes exposure to their environment, and may increase tolerance of environmental extremes (Guidetti et al., 2011).

Figure 1: Size comparisons of different tardigrade species. Lengths and widths of tardigrades were measured via FIJI/ImageJ from journal articles describing each species shown.
Though tardigrade species can differ in overall appearance, they all share a segmented body plan, with a head and four pairs of lobe-like legs that end in claws (Figure 2A, Nelson et al., 2015). The first three pairs of legs are used in locomotion, while the fourth pair of legs face rearward, providing grip and stability. Even with all these legs, tardigrades move quite slowly; in fact, the name ‘tardigrade’ means ‘slow walker’. Their lumbering gait and stocky body makes them reminiscent of little bears or pigs, giving them their common names of ‘water bears’ or ‘moss piglets’. They are especially cute and teddybear-like when they have eyespots, which are prominent in some (but not all) species of tardigrade (Figure 2B).
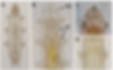.png)
Figure 2: Representative images of tardigrades found in the summer of 2020. A. Ventral (belly) view of a tardigrade, head towards the top. Note the head, the three pairs of legs along the body, and the fourth pair of legs that point rearward. B. Ventral view of the anterior portion of a tardigrade with eyespots (ey) and storage cells (sc) labeled. C. Ventral view of the head, showing sensory bristles. D. Ventral view of the trunk, showing bands of muscles.
Because of their small size, tardigrades do not require a respiratory system to facilitate gas exchange, nor do they need a circulatory system to transport nutrients and gases around their bodies (Nelson et al., 2015). They do, however, have organ systems dedicated to digestion & excretion, sensation & movement, and reproduction.
Digestion: Tardigrades feed on the mosses, algae, and other plant matter that they live amongst and on, although some larger species of tardigrades eat other animals such as rotifers or nematodes (Figure 3). Many tardigrades are ‘suctorial’ feeders: they use sharp stylets to pierce their food, and suck out the good stuff. Food travels through their mouth and buccal tube into a muscular chamber called a pharynx, which is flanked by a pair of salivary glands. Food then travels through a short esophagus to the midgut and the hindgut of the tardigrade (Gross et al., 2015). Near the boundary between the midgut and the hindgut, a system of Malphigian tubules is attached to the digestive tract (Gross et al., 2019). These structures are thought to act in excretion and osmotic balance (Gross et al., 2015). Waste then exits the body through a cloaca (Figure 4).

Figure 3: Tardigrade friends … and sometimes food. A. nematode (roundworm) found in the same moss sample as a tardigrade. B. rotifer, found in the same sample.
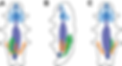
Figure 4: Tardigrade reproductive and digestive systems. (A) dorsal view (B) lateral view (C) ventral view. Ovary and eggs (green), mouth (light blue) connected to the salivary glands (light blue), pharynx (royal blue), malpighian tubules (orange), cloaca (dark navy blue), gut (purple).
Reproduction: Tardigrades show a wide range of reproductive strategies (Bertolani, 2001). Some species, like the commonly-used lab species Hypsibius exemplaris, are parthenogenetic and are all female. Other species have separate sexes, or are gonochorous; this this 2-sex situation is especially prevalent in marine species. Still other species are hermaphroditic. and have both female and male gonads, but hermaphroditism appears to be rare amongst tardigrades (Nelson et al., 2015). The ovary of female tardigrades is located dorsal and just a bit lateral to the midgut (Gross et al., 2019). In males, a pair of sperm ducts empties into the cloaca (Gross et al., 2015).
​
Tardigrades are members of the same clade (Ecdysozoa) as two widely-used model organisms: Drosophila melanogaster, and Caenorhabditis elegans. These well-studied organisms exhibit strikingly different mechanisms of sex determination and dosage compensation. It would be interesting to learn how sex is determined in gonochorous species of tardigrades, whether sex chromosomes exist in these species, and if so, how chromosome dosage is accomplished.
Movement: Tardigrades have bands of muscle that are readily visible under standard light microscopy (Figure 2D). These muscles are attached to the inner cuticle, and stretch across or along the length of the tardigrade’s body (Figure 5). Each pair of legs is associated with a ganglion, or group of neuronal cell bodies, that presumably regulate locomotion. These ganglia are located along the ventral side of the body, and are connected by two tracts of fibers that run in parallel. The parallel fiber tracts continue towards the head, where they connect with the dorsally-located brain (Nelson et al., 2015). Some tardigrade species can sense light via eyespots; in addition, some tardigrade species interact with their environment via sensory projections that emanate from their heads like antennae (Figure 2C), or via similar spiky appendages along their bodies (Gross et al., 2015).

Figure 5: Tardigrade muscles and nerves.
Several questions arise about how the nervous system functions in tardigrade locomotion. For example, how do the tardigrades coordinate their leg movements? Does each leg move independently of its pairmate, or do they move alternately under control of a pattern generator? Does each pair of legs move independently of the other pairs? Do tardigrade neurons have action potentials like their Panarthropod relative Drosophila, or is neuronal spiking mostly absent or rare, as in their Ecydosozoan relative C. elegans (Liu et al., 2018).
Like all animals, tardigrades need mechanisms to convert food into energy, to move, and to reproduce, so the internal organ systems described so far are familiar to us. Two features of internal tardigrade anatomy that are less familiar are the claw glands and the storage cells (Gross et al., 2019). Claw gland cells are straightforward: they release materials to grow claws. The storage cells are a bit more mysterious (Figure 2B). Their number and size varies greatly between tardigrade species. They are distributed throughout the body, and may have roles in nutritional maintenance, egg formation, and perhaps immune function (Gross et al., 2019).
Like C. elegans, tardigrades have been described as eutelic, which means that they may have a stereotyped number of somatic cells when adults. The eutelic status of tardigrades, however, has been recently shown to be not quite true. Some cells - especially the storage cells - do divide in adult animals (Czernekova & Jönsson, 2016). Nevertheless, the small size and relatively small number of cells in adult tardigrades may enable it to be a developmental model comparable to C. elegans (Gabriel et al., 2007). Indeed, Sydney Brenner considered tardigrades when he was searching for a model of nervous system development and function (Gabriel et al., 2007). Tardigrade adults have more neurons than adult C. elegans, and this difference in neuron count reportedly tipped the balance in favor of the worm.
​
Several questions need to be addressed to evaluate the potential of tardigrades as a model system for development. For example, does each cell in an adult tardigrade have a specific, stereotyped identity and function, invariant from animal to animal? Is there an invariant cell lineage during development? Analysis of the first ~7 divisions in Hypsibius dujardini suggests that cleavage patterns and migration of nuclei and cells occur reproducibly across individuals, at least during the earliest stages of development (Gabriel et al., 2007). Can the function of individual identified cells be determined via targeted laser or genetic ablation, as in C. elegans (Bargmann & Avery, 1995)? Can we map all of the synapses in a tardigrade? Clearly there is much to learn about tardigrade development!
References:
​