Development and Embryogenesis
Embryogenesis & Development in Tardigrades
The study of how a single sperm and egg grow into an adult organism is called embryology and developmental biology. A fundamental question in these fields is how the cells of multicellular organisms differentiate; how each cell decides to become part of the brain, leg, or another organ. Tardigrades, microscopic “water bears” that live in moss and lichen, are animals that can help answer these questions. There are over a thousand species of tardigrade on the planet that each undergo reproduction, embryogenesis, and development in unique ways. Three major reproductive patterns can be found. Gonochoristic (individuals are either male or female), hermaphroditic (an individual can be both male and female), and parthenogenetic (eggs can develop without being fertilized) species of tardigrade have been found. Researchers have also observed tardigrades that appear to reproduce by internal fertilization. (Poprawa and Janelt 2019). This diversity of reproductive strategies makes the tardigrade a promising model organism for answering the questions of developmental biology.
There are four main stages of embryogenesis: oogenesis, previtellogenesis, vitellogenesis, and choriogenesis. Oogenesis is the process in which an egg cell becomes a mature ovum ready for fertilization. In tardigrades, cells called “germ cells”, capable of making eggs or sperm, are found in clusters. Only one cell in the cluster is destined to differentiate into an oocyte, or a premature egg, while the rest of the cells will become trophocytes, cells that provide nourishment for the growth of the oocyte cell (Poprawa and Janelt 2019). The second stage is previtellogenesis. During this time, the egg makes RNA, ribosomes, and cell organelles. Interestingly, it seems that these structures are transported between the cells of the cluster. Organelles have been found in cytoplasmic bridges connecting cells together, suggesting that the cells share these resources (Poprawa and Janelt 2019).
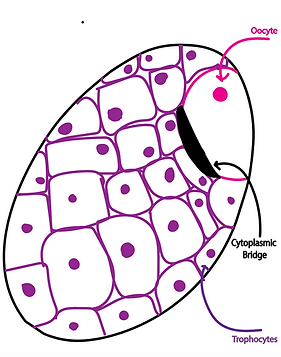
Cytoplasmic Bridges
Cytoplasmic Bridges, shown in black, allow trophocytes, or polar bodies, to share their nutrients with the oocyte. This allows the trophocytes to takeover the job of producing nutrients for the oocyte.

Oogenesis
During meiosis a gamete (egg or sperm cell) divides into four cells. Eggs convert three of those cells into polar bodies through the process of oogenesis. The polar bodies serve to nourish the remaining cell, which becomes a mature oocyte (egg).
During vitellogenesis, the trophocytes dump nutrients into the oocyte to form the egg yolk. The yolk, like in a chicken egg, is a collection of nutrients that the developing embryo uses for energy and building materials. Vitellogenesis has three stages: early, middle, and late. During early vitellogenesis all of the cells in the cluster begin accumulating yolk materials and are the same shape and size. Middle vitellogenesis starts when one cell in the cluster begins to grow more rapidly than the others. This is the cell that differentiates into the oocyte while the others become trophocytes. Nutrients synthesized by the trophocytes are transported to the oocyte via the cytoplasmic bridges. During late vitellogenesis the oocyte packs these nutrients to form the yolk (Poprawa and Janelt 2019). Lastly, choriogenesis is the process in which the chorion, or eggshell, forms. Tardigrade egg shells form two layers, a thin envelope that sticks to the egg and a thicker protective shell (Poprawa and Janelt 2019).
Once the fully developed egg is fertilized by sperm, it starts to develop into a baby tardigrade. The growing tardigrade needs to determine its body plan, or what end is the head, what end is the butt, and where the legs will be. One example of this planning is in the development of the central nervous system. As the nervous system develops, neurons are growing in the right numbers, moving to the right places, and making the right connections to each other in a complicated dance to form the complex structures of the brain and spinal cord. The tardigrade central nervous system is organized into segments. The first signs of nervous system development are clusters of neurons called ganglia, which can be seen thirty-five hours after the egg is laid (Smith, Cumming, and Goldstein 2018). The first ganglion to develop is found in the head. These first neurons eventually become the brain and the precursor to the mouth, called the stomodeal compex. As the brain develops, the central commissure, or nerve tissue that connects the two sides of the brain, thickens as more cells grow on its upper side. A pair of neurons also grow on the underside of the brain. These cells all become connected to the spinal cord (Gross and Mayer 2015). Only ten hours later, the central nervous system appears to be fully developed (Smith, Cumming, and Goldstein 2018).
​
The formation of these complex structures is largely ruled by expression of different genes that tell cells how to grow. There are several tardigrade genes involved in the processes of nervous system development, including He-elav, He-six3, and Pax3/7. He-elav is heavily concentrated in areas where the central nervous system develops. He-elav codes for a family of splicing factors, proteins that process RNA to become specific proteins that are only found in neurons. He-six3 is expressed during all developmental periods of the tardigrade. Early in development, it was found primarily in the developing head, and later it was found specifically in the developing inner brain and stomodeal complex (Smith, Cumming, and Goldstein 2018). Pax3/7 can be detected in the front end of the developing tardigrade in the head and mouth precursors. It is believed that Pax3/7 could localize specifically in cells that will become neurons (Gabriel and Goldstein 2007). The high concentrations of these genes during certain stages of development indicate to the cells how to differentiate, and thus how to build the nervous system in an orderly fashion. Similar processes are happening all over the developing tardigrade to build each organ system.
Works Cited
Gabriel, Willow N., and Bob Goldstein. 2007. “Segmental Expression of Pax3/7 and Engrailed Homologs in Tardigrade Development.” Development Genes & Evolution 217(6): 421–33.
​
Gross, Vladimir, and Georg Mayer. 2015. “Neural Development in the Tardigrade Hypsibius Dujardini Based on Anti-Acetylated α-Tubulin Immunolabeling.” EvoDevo 6(1): 12.
​
Poprawa, Izabela, and Kamil Janelt. 2019. “Reproduction, Gonad Structure, and Oogenesis in Tardigrades.” In Evo-Devo: Non-Model Species in Cell and Developmental Biology, Results and Problems in Cell Differentiation, eds. Waclaw Tworzydlo and Szczepan M. Bilinski. Cham: Springer International Publishing, 495–513. http://link.springer.com/10.1007/978-3-030-23459-1_20 (June 2, 2020).
​
Smith, Frank W., Mandy Cumming, and Bob Goldstein. 2018. “Analyses of Nervous System Patterning Genes in the Tardigrade Hypsibius Exemplaris Illuminate the Evolution of Panarthropod Brains.” EvoDevo 9(1): 19.